Drones – from delivery and surveillance to commuting
In 1935, the DH.82 Queen Bee, a pilotless, radio-controlled variant of the de Havilland Tiger Moth, became available for use in training anti-aircraft gunners ; its name is widely believed to explain why today’s UAVs are often called ‘drones’.
Since those early days, drones have not only found important military roles, but are also becoming increasingly powerful tools for government, commercial and consumer applications. While they are currently deployed for military, visual or cargo payload roles, Uber and other companies are planning to introduce drones designed for passenger transportation.
This article reviews present and future drone technology. We look at the diversity of applications where they’re currently used, and discuss the onboard technologies that take them in to these. We also address national and international legislation that impacts drones. We then consider how drones can scale up to passenger transport, and the new set of challenges that this presents.
Drone applications
Drones can perform air operations that manned aviation finds difficult or impossible, and they provide evident economic savings and environmental benefits whilst reducing the risk to human life. They already surpass manned aircraft in endurance, range, safety and cost efficiency, but next-generation devices will widen the gap still further, adding greater stealth, sensory, payload, range, autonomous and communications capabilities. Drone sensor technology currently in development can map 2.7 million square miles in a single flight – nearly the area of the 48 contiguous US states.
With their growing capabilities, drones are being used in an ever-wider and more innovative range of applications, each with their own unique set of challenges. While some drones are versatile enough to fulfil many types of mission, most are more specialised, and designed for a single purpose.
Typical applications include:
- Agriculture
- airborne early warning
- anti-submarine
- cargo transport
- communication relay
- conservation
- decoy
- disaster recovery
- environmental monitoring
- fire fighting
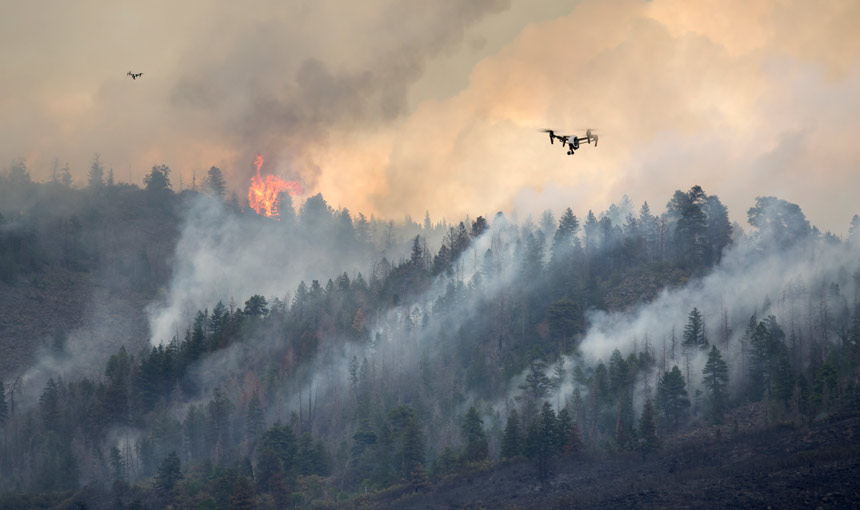
Fig.1: Drones with cameras can detect and inform on fires early
- intelligence
- meteorology
- mine detection and detonation
- natural resources
- personnel transport
- photography
- pipeline inspection
- reconnaissance
- remote sensing
- search
- surveillance
- surveying
- target designation
- traffic
- wildlife monitoring
Drone applications – operational considerations
Drones are available in many types of geometries, which can be broadly classified under three headings: Fixed wing, helicopter and multirotor. The choice of geometry, and the best way of assembling and equipping it, depend on the application. Below are some factors to consider when choosing a drone solution, as described in an article by CMEC, who are designers and manufacturers of batteries for electric UAVs.
Multirotor drones are an easy and cheap way of getting a small camera into the air for a short time. They allow good control over position and framing, making them perfect for aerial photography work. However, they have limited endurance and speed, making them unsuitable for aerial mapping, long endurance monitoring and long-distance inspection as needed for pipelines, roads, and power lines.
Although their technology is continually improving, multirotors are fundamentally inefficient and energy-hungry, as they must constantly fight gravity just to stay aloft. Current battery technology limits electric drones to around 20 – 30 minutes’ flight time with a lightweight camera payload. Internal combustion (IC) engines aren’t suitable, as they cannot handle the fast and high-precision throttle changes essential for stability.
By contrast, fixed-wing drones, like aeroplanes, use their wings rather than vertical rotors for lift. Requiring energy only to move forward, they are far more fuel-efficient than multi-rotors. Additionally, these drones can use IC engines, so many can stay aloft for up to 16 hours. They can cover longer distances, map much larger areas, and loiter for extended periods monitoring their point of interest.
However, fixed wing drones also have downsides. Being unable to hover, they’re unsuitable for aerial photography work. Launching and landing is more demanding, as they need a runway or catapult for take-off and a runway, parachute or net to land safely. Only the smallest fixed-wing drones are suitable for hand launch and ‘belly landing’ in an open field. These types are also higher-cost, and operating them is more difficult to learn.
Single-rotor helicopters are another option. While a multi-rotor drone has many rotors to keep it aloft, a helicopter has just one, plus a tail rotor to control its heading. Helicopters are more efficient than multi-rotors, and, for even better endurance, can use IC engines for propulsion. One rule of aerodynamics is that increasing size and reducing spin speed improves rotor blade efficiency; this is why a quad-copter is more efficient than an octo-copter, and special long-endurance quads have a large prop diameter. A single-rotor helicopter allows for very long blades which are more like a spinning wing than a propeller, giving great efficiency.
Single-rotor helicopters are ideal for hovering with a heavy payload such as an aerial LIDAR laser scanner, or for hovering combined with long endurance or fast forward flight. The downsides are their complexity, cost, vibration, and the danger of their large spinning blades, which represent a significant safety hazard. They can hover on the spot, making them easy to learn and manage, but they’re not as stable or forgiving of bad landings as multirotors. Additionally, their mechanical complexity demands much maintenance and care.
A new alternative, called fixed-wing hybrid VTOL, merges the benefits of fixed-wing flight with an ability to take off and land vertically, and hover. These concepts were tried out with manned aircraft in the 50s and 60s, but proved too complex and difficult to fly. However, they are now beginning to become feasible, with the arrival of modern autopilots, gyros and accelerometers. The autopilot can maintain stability, leaving the human pilot the easier task of guiding them around the sky.
The relative merits of each drone type are summarised in the table of Figure 2.
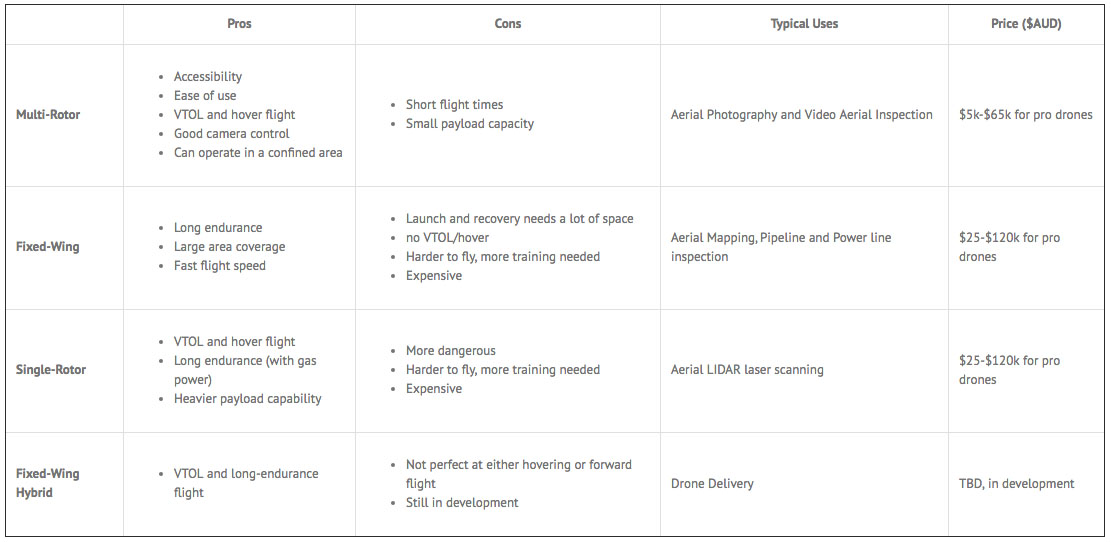
Fig.2: Drone type comparison table – Image via Australian UAV
Drone legislation
Legislation as well as technology is a limiting factor on drone industry growth. Uncertainty weighs on innovation and commercial adoption, but anticipated regulatory clarity should unlock demand. NASA is leading a multibillion-dollar effort to develop a US airspace management system capable of safely coordinating manned and unmanned flight, while the Federal Aviation Administration (FAA) is expected to further ease restrictions that are keeping commercial drones from reaching their full potential.
Current FAA regulations restrict drones from flying over 400 feet, and they must stay within their pilot’s line of sight. Autonomous operation is not allowed. They cannot operate over people, and must be flown by someone with a remote pilot certificate.
In Europe, the European Aviation Safety Agency (EASA) has published an Opinion 01/2018 which includes a proposal for a new regulation for UAS operations, with three categories:
- open’ category is a category of UAS operation that, considering the risks involved, does not require a prior authorisation by the competent authority nor a declaration by the UAS operator before the operation takes place;
- ‘specific’ category is a category of UAS operation that, considering the risks involved, requires an authorisation by the competent authority before the operation takes place, considering the mitigation measures identified in an operational risk assessment, except for certain standard scenarios where a declaration by the operator is sufficient or when the operator holds a light UAS operator certificate (LUC) with the appropriate privileges;
- ‘certified’ category is a category of UA operation that, considering the risks involved, requires the certification of the UAS, a licensed remote pilot and an operator approved by the competent authority, in order to ensure an appropriate level of safety.
Each European country has its own aviation authority in addition to any applicable European legislation. In the UK, this role is fulfilled by the Civil Aviation Authority (CAA), whose aim is to ensure that all Unmanned Aircraft Systems (UAS) are fully and safely integrated into the UK’s total aviation system. The type and extent of CAA regulations imposed on a drone depend on the following parameters :
Type of operation: Within the Visual Line of Sight (VLOS) or beyond the Visual Line of Sight (BVLOS) of the person flying the aircraft
Purpose of flight: Recreational, Commercial or Private/Non-Commercial
Weight (Mass) category:
- 20 kg or less (Small unmanned aircraft) Small Unmanned Aircraft - this class covers all types including traditional remotely controlled model aeroplanes, helicopters or gliders, as well as the increasingly popular multirotor ‘drones’ and remotely controlled ‘toy’ aircraft. They normally have a reduced level of regulation imposed on them which is aimed at being proportionate to the risk and complexity or their types of operation.
- 20 – 150 kg (Light unmanned aircraft) this class covers the larger and potentially more complex types of unmanned aircraft and large model aircraft. They are subject to all aspects of UK aviation law, although it is accepted that they will require to be exempted from many of the requirements.
- Over 150 kg (UAS) Unmanned aircraft within this class will normally be subjected to the same level of regulatory approval requirement as would be used for traditional manned aircraft. They will normally be certificated by the European Aviation Safety Agency (EASA).
New UK Government laws, introduced in May 2018, are intended to tighten the safety of all drone flights . All drones are restricted from flying above 400 feet and within one kilometre of airport boundaries. Additionally, drone users have to register and take online safety tests to improve accountability.
Commuter and air taxi passenger drones
With progress in legislation and the above technologies, a $100 billion market opportunity for the period 2016 - 2020 has been forecast for drones in military, payload and vision applications. However, many entrepreneurs and engineers around the world are pushing for a yet more exciting development; using drones for passenger transportation. In and around most large cities, ever-growing traffic volumes are making ground-based commuting and taxi transport an increasingly frustrating and time-consuming experience. Wouldn’t it be great if we could relieve this with drones and their access to three-dimensional space?
To make this a viable and safe proposition requires considerable further development of drone technology, legislation and infrastructure – but progress is being made, and detailed plans are being developed to overcome these challenges. In September 2017, Dubai conducted its first test of a drone taxi service that it hopes will become a viable transportation system in the city. The test comprised a two-seater, 18-rotor Volocopter drone which completed a five-minute flight above a strip of sand on the Gulf coast.
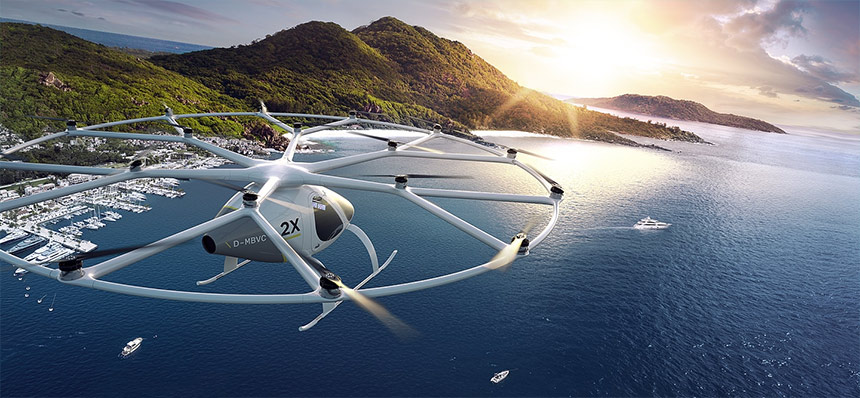
Fig. 3: Volocopter passenger drone – Image via Volocopter
Volocopter, the drone’s designer, hopes to have the taxis up and running within four years. "Implementation would see you using your smartphone, having an app, and ordering a Volocopter to the next Voloport near you," said chief executive Florian Reuter.
"The Volocopter would come and autonomously pick you up and take you to your destination."
A number of other companies, including Airbus and several of its subsidiaries, are researching self-flying drone technology. Chinese company Ehang has launched their 184 , which can carry a single passenger for up to 23 minutes on one battery charge, giving a flight radius of around 10 miles.
Uber is developing plans, under an initiative they call ‘Uber Elevate’ , to develop passenger drone technology and the infrastructure to support an air taxi drone service.
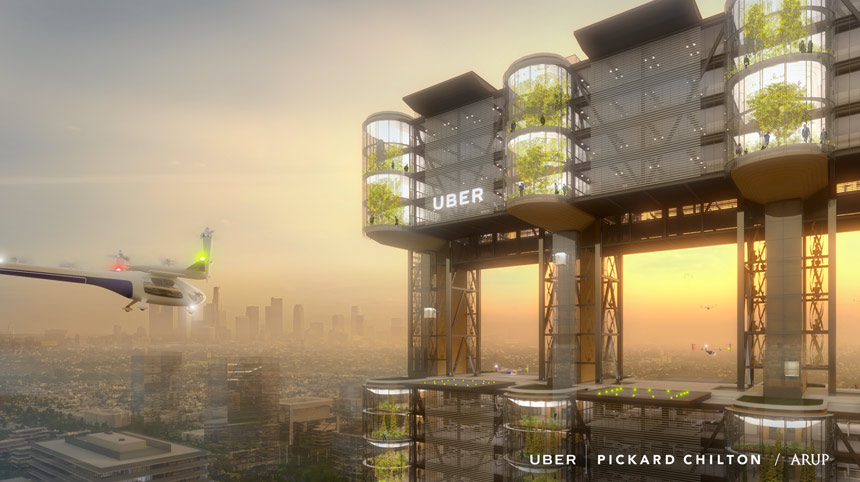
Fig.4: Uber drone and vertiport – Image via Uber
The concept is based on a network of small, electric aircraft that take off and land vertically (VTOL) that will enable rapid, reliable transportation between suburbs and cities, and, ultimately, within cities.
The VTOL aircraft could be complemented by an urban VTOL infrastructure of ‘vertiports’ based on the repurposed tops of parking garages, existing helipads, and even unused land surrounding highway interchanges. These vertiports would include multiple take-off and landing pads as well as charging facilities. The infrastructure could also contain single-aircraft ‘vertistops’ with minimal facilities.
Uber believes that in the long term, VTOLs will become an affordable form of mass daily transportation, even less expensive than car ownership. If VTOLs serve the on-demand urban transit case well, with quiet, fast, clean, efficient and safe transportation, then a path to high production volumes and associated lower per-unit costs may exist. This path can be helped, especially initially, with the ridesharing model and its amortisation of costs.
Success in this ambitious vision depends on effective co-operation between the key players within the VTOL ecosystem – regulators, vehicle designers, communities, cities, and network operators. Uber has identified that bringing on-demand urban air transportation to market depends on resolving the following critical challenges:
The certification process: Before VTOLs can operate in any country, they will need to comply with regulations from aviation authorities—namely the US Federal Aviation Administration (FAA) and European Aviation Safety Agency (EASA) who regulate 50% and 30% of the world’s aviation activity.
Battery technology: Electric propulsion has many desirable characteristics that make it the preferable propulsion choice for the VTOL aircraft as discussed, and batteries are the obvious energy source. However, improvements in several key areas are needed to improve their viability; energy per unit weight, charge rate, cycle life, and cost per kWh. A collaboration between the US Department of Energy and university labs is focusing on lithium-metal solutions. There is also research into pulse chargers which could significantly reduce charging times.
Vehicle efficiency: Helicopter rotor flight is not as efficient as fixed-wing operation, due to wing lift efficiency. Efficiency must be improved to make VTOL aircraft commercially viable.
Vehicle performance and reliability: vehicle performance relates to cruise speed and take-off and landing time, and system reliability is measured as time from request until pick-up. Robustness in varied weather conditions is also a concern, as bad weather could ground a large percentage of a vehicle fleet at arbitrary times.
Air traffic control: Urban airspace is actually open for business today, and with ATC systems exactly as they are, a VTOL service could be launched and even scaled to possibly hundreds of vehicles. Under visual flight rules (VFR), pilots can fly independent of the ATC and when necessary, they can fly under instrument flight rules (IFR) leveraging existing ATC systems. A successful, optimized on-demand urban VTOL operation, however, will necessitate a significantly higher frequency and airspace density of vehicles operating over metropolitan areas simultaneously. In order to handle this exponential increase in complexity, new ATC systems will be needed. Uber envisions low altitude operations being managed through a server request-like system that can deconflict the global traffic, while allowing UAVs and VTOLs to self-separate any potential local conflicts with VFR-like rules, even in inclement weather.
Cost and affordability: Helicopters – the closest practical proxy to the proposed VTOL solutions, are too expensive and noisy for large-scale use in urban areas. Uber proposes simpler, quieter and more operationally efficient vehicle designs which leverage digital control rather than mechanical complexity. This could kickstart a virtuous cycle of economies of scale providing cost and price reduction.
Safety: Uber describes plans to ensure VTOLs are twice as safe (half the fatalities) as privately-operated cars.
Aircraft noise: Electric propulsion will be critical in achieving noise levels low enough to be acceptable to any affected community.
Emissions: Electrically propelled vehicles have zero operational emissions. However, this still leaves concerns about how the electricity for recharging the VTOL batteries is generated; currently, this is still largely coal, natural gas and petroleum based. Vertiport/vertistop infrastructures in cities: The greatest operational barrier to deploying a VTOL fleet in cities is a lack of sufficient locations to place landing pads that are readily accessible and can support charging stations. The Uber report discusses how infrastructure could be developed to overcome this problem.
Pilot training: Training to become a commercial pilot is demanding and time-consuming, and a shortage of qualified pilots is expected to curtail growth significantly. Pilot augmentation technology will significantly reduce pilot skill requirements, and this could lead to a commensurate reduction in training time.
VTOL possibilities - NASA and FAA views
NASA and the FAA recently spearheaded a series of On-Demand Mobility (ODM) workshops to bring the VTOL ecosystem together in identifying barriers to launching an on-demand VTOL service. Their findings aligned quite well with Uber’s.
How to make a drone
If you’re interested in constructing your own drone, Coleman Benson has prepared an 8-lesson tutorial explaining how to do so; this can be found on the RobotShop website . Below is a summary of the topics covered:
Terminology: The tutorial starts with a Terminology reference list, including definitions of various UAV geometries.
The frame: The first step in UAV construction is to choose the frame; this can be either assembled from a UAV frame kit, or entirely DIY. Geometry options include:
- Tricopter: Three arms each with one motor
- Quadcopter: Four arms each with one motor
- Hexacopter: Six arms each with one motor
- Y6: Three arms, each with two motors, one top and one bottom. Note that all propellers project thrust downwards.
- Octocopter: Eight arms each with one motor
- X8: Like a Y6, but with four arms, each with two motors
Size must also be decided. For hobbyists, the best size range for versatility and value is between 350 mm and 700 mm, as a measurement of the diameter of the largest circle that intersects all of the motors. Decisions between construction materials – wood, foam, plastic, aluminium and others – must also be made.
Other considerations include:
- A gimbal, for mounting and stabilising cameras
- Payload: the extra weight must be accounted for, and the load must be secured against shifting in flight.
- Landing gear
- Mounting
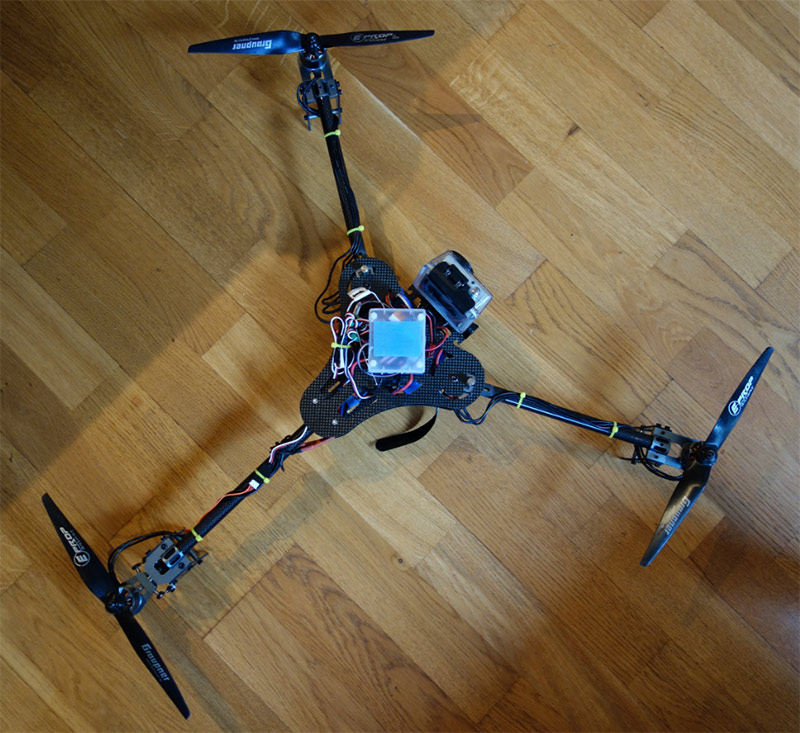
Fig.5: Tricopter – Image via flickr
Propulsion: A complete propulsion system includes motors, propellers, electronic speed controllers (ESCs) and a battery. Almost all small multi-rotor drones / UAVs are electric, with almost none being gas-powered.
Brushless DC motors are used extensively in the hobby RC industry for products ranging from helicopters and aeroplanes to the drive system in RC cars and boats. Smaller UAVs (usually the size of the palm of your hand) tend to use small brushed motors because of the lower price and simpler two-wire controller. Key parameters for motors include KV ratings (rotation speed for a given voltage) and thrust in Kg, Lb or N.
Propeller parameters include number and diameter of blades, pitch, angle of attack, efficiency and thrust, rotation (clockwise or anti-clockwise), material, folding, mounting, prop savers and guards, and balancing.
An ESC (acronym for “Electronic Speed Controller“) controls the speed and direction of a motor. The ESC must be able to handle the maximum current which the motor might consume, and provide it at the right voltage.
Most batteries are lithium polymer (LiPo), or possibly lithium-manganese or another lithium variant. LiPo offers high capacity with low weight, together with high discharge rates. However, they are of comparatively higher cost, and have some safety issues.
Flight controller: A flight controller for a multi-rotor UAV is an integrated circuit normally made up of a microprocessor, sensors and input / output pins. Out of the box, a flight controller does not magically know your specific UAV type or configuration, so you need to set certain parameters in software, and once complete, that configuration is then uploaded to the board.
Multirotor UAV flight controllers will be connected to the sensors listed below, or a subset of them.
- Accelerometer: measures acceleration in up to three axes and helps maintain UAV stability
- Gyroscope: measures the rate of angular change in up to three angular axes
- Inertia measurement unit (IMU): a small board which contains both an accelerometer and gyroscope. It may contain additional sensors such as a three-axis magnetometer.
- Compass/magnetometer: An electronic magnetic compass measures the earth’s magnetic field and uses it to determine the drone‘s compass direction (with respect to magnetic north). This sensor is almost always present if the system has GPS input.
- Pressure/barometer: Provides accurate reading of UAV height
- GPS: Uses the signals sent by satellites in orbit around the earth to determine the UAV’s specific geographic location
- Distance sensors: Measures distance from the ground – or a hill, mountain or building – to minimise risk of hitting a target. Distance sensors use Lidar, laser or ultrasonic technology.
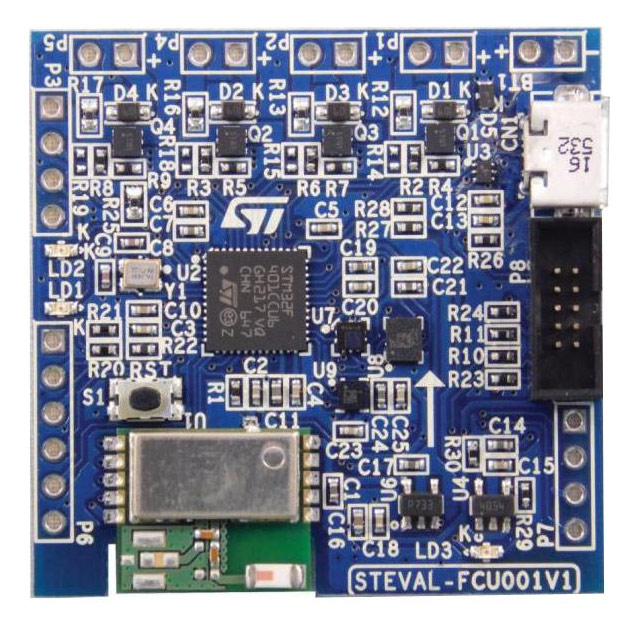
Fig.6: STEVAL-FCU001V1 Evaluation Board , Complete Flight Controller Unit for Toy Drones, Flight Sensors, Bluetooth - available from Farnell
A remote control (RC) transmitter and receiver is needed to allow an operator to control the drone. The (usually) hand-held transmitter has at least four channels, comprising:
- Pitch (which translates to forward / backward motion)
- Elevation (closer to or farther away from the ground)
- Yaw (rotating clockwise or counter-clockwise)
- Roll (to strafe left and right)
Additional channels can include:
- Arming / disarming the motors
- Gimbal controls (pan up/down, rotate clockwise / counter-clockwise, zoom)
- Change flight modes (acrobatic mode, stable mode etc)
- Activate / deploy a payload, parachute, buzzer or other device
- Any number of other uses
Assembly: The Assembly tutorial contains practical information, and mistakes to avoid, when assembling the frame, motors, ESCs, propellers, battery, charger, power distribution, flight controller and communications device.
Get it all working together: Setting up and configuring the assembled components
First Person View (FPV) and long range: This tutorial introduces the concepts of First Person View (FPV) and long-range control. FPV currently involves mounting a video camera on the drone which sends video in real time to the pilot. FPV systems can work with 3D/VR head mounted displays from Occulus Rift, Samsung, Gear, Morpheus and other manufacturers.
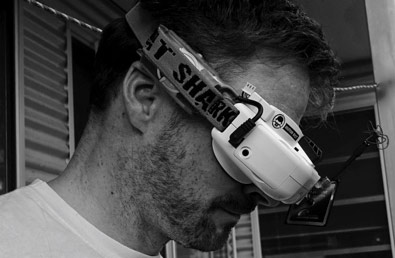
Fig.7: Drone FVP headset – Image via Pixabay
Aircraft: For some applications, a fixed-wing design might be preferable to a multirotor approach. This tutorial compares the two design geometries.
Summary and conclusion
This article has introduced the concepts of UAV operation and construction, and looked at their existing and potential applications. This has included a closer investigation of the exciting and somewhat – but not entirely – futuristic possibilities and challenges of manned UAVs.
We finish with a summarised listing of all the factors relating to UAVs and their flight. It has been beyond the scope of this article to cover all these in depth, but the listing should serve as a basis for further research. It’s based on an article titled ‘Drone Technology’ written by Ian Burns of ATIP Law.
Airframes and Structure
- endurance
- launch and recovery
- manoeuvrability
- on-board systems
- operating altitude
- operating environment
- range
- scale effects
- stability
- stealth
- transportation and storage
Construction methods
Propulsion systems
- control
- endurance
- maintenance
- monitoring
- size and weight
Power systems
Stealth
- electromagnetic emissions
- infrared
- radar
- sound
- visible
Launch and recovery systems

Fig. 8: Scan Eagle Catapult – Image via Wikipedia
Communication systems
Control systems
- acceleration and vibration tolerant
- interference
- moisture
- power conditions
- temperature range and thermal cycles
Collision avoidance
- communicating with air traffic control, other aircraft and ground stations
- sensing other traffic
- determining potential collisions
- taking evasive action
Data
- acquisition
- processing
- storage
- transmission
Support
- diagnostics
- mission planning
- recharging
- refuelling
- repair and maintenance
- training
- security
References
https://www.dehavillandmuseum.co.uk/aircraft/de-havilland-dh82b-queen-bee
https://www.goldmansachs.com/our-thinking/technology-driving-innovation/drones/index.html
https://www.auav.com.au/articles/drone-types
https://www.easa.europa.eu/easa-and-you/civil-drones-rpas
https://www.gov.uk/government/news/new-drone-laws-bring-added-protection-for-passengers
https://www.bbc.co.uk/news/technology-41399406
https://ttalents.com/ehang-autonomous-aerial-vehicle
https://www.uber.com/elevate.pdf
http://www.nianet.org/ODM/roadmap.htm
https://www.robotshop.com/blog/en/make-uav-lesson-1-platform-rtf-arf-kit-custom-13989
https://atintellectualproperty.com/drone-technology
Drones – from delivery and surveillance to commuting - Date published: 15th October 2018 by Farnell element14